Ancient Mojave Watershed Project
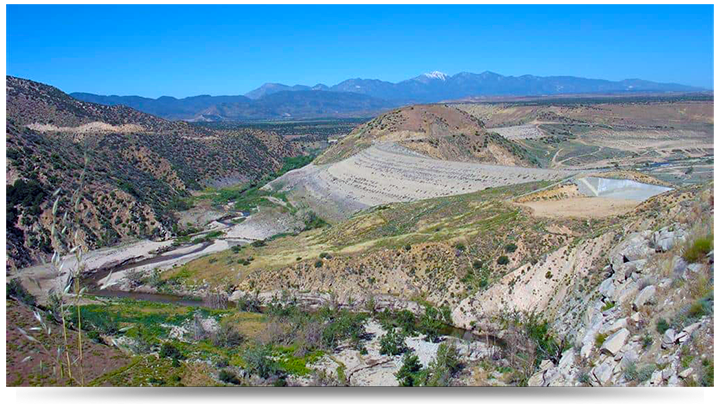
Photo; view from Deep Creek Mojave River source looking westward to wards the Mojave Dam
and beyond the western source coming from present day Lake Silverwood.
and beyond the western source coming from present day Lake Silverwood.
The goal is to explore the Ancient Mojave river and corresponding lake systems accessing material from six areas of science and connected sub-studies.
Many days and nights were spent at Camp Leakey looking east over the Mojave River Valley towards distant Newberry Springs and the immensity of ancient Lake Manix that once filled it. Gradually, questions were formulated concerning the processes and forces that formed Lake Manix and the string of other lakes in the region. Also, of course, what were the possible animal and eventual human dates and interactions in the margins of the river and lake systems.
This space will provide a few basic facts beginning with the basic timeline and physical pieces of the puzzle that together produced the Mojave River watershed. The main physical entities will be identified and described in basic language. Much of the material will be used initially from Wikipedia and will contain attribution links with Wikipedia References and other sources.
There is a wide variety of material available for the southern Mojave. The story will continue to advance and include material for more advanced studies in at least six main fields; anthropology, archaeology, paleontology, geology, geography, hydrology. Of course, as many sub-divisions of each of the listed fields that are relavant will be included of each of the listed fields that are relavant will be included with proper attribution. New visual materials will be created too, to enhance our descriptions.
Though I was a dual major at University of California, Riverside, in the late 1970s, anthropology and studio art. I pursued teaching as my profession and my art as my occupation. I taught elementary school for fourteen years and then secondary for another fourteen. But, I have always been an advocate of the sciences involved with this project. The purpose of this project is to tell a story, to accomplish this I must gather general and specific information and make it available in an easy to follow format.
~ The Mojave River Project Begins with Three Important Questions ~
As the Transverse Range developed the primary drainage for the central region was to the south into the Santa Ana River drainage. What caused the drainage to be re-directed north into the Mojave River drainage?
Traverse Range Drainage Info
What were the mechanisms that allowed vast amounts of water to be deposited as snow and rain in the Transverse Range and retained extensively in the Mojave River string of lakes and elsewhere in the Mojave and Great Basin?
Traverse Range Precipitation info
Lake Manix water level was 800 feet higher than Lake Mojave (1780 vs 940). How did Lake Manix breech location of Afton Canyon to reach Lake Mojave? How high was the maximum level of Lake Manix to allow for the breech to occur?
Manix Afton Canyon formation Info
Included below are basic information that will orient the reader with the foundational facts; Pleistocene and Holocene epochs, San Andreas Fault, Transverse Ranges, Pleistocene Glaciation, Mojave River and Associated Lakes.
~ The Pleistocene Epoch ~
Pleistocene and Holocene Epochs
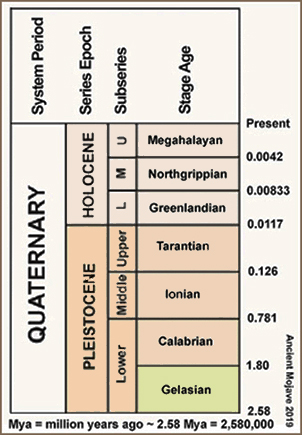
The information above is generally agreed on, though there are several differing views. In any case, this is what our project will use as age references.
Our project will begin with the changes and development of the Mojave watershed during the Pleistocene. These changes occurred along the transverse range immediately to the south and the San Andreas fault system which runs parallel and crosses the Transverse range.
The Pleistocene is the geological epoch which lasted from about 2,588,000 to 11,700 years ago, spanning the world's most recent period of repeated glaciations. The end of the Pleistocene corresponds with the end of the last glacial period and also with the end of the Paleolithic age used in archaeology.
The Pleistocene is the first epoch of the Quaternary Period or sixth epoch of the Cenozoic Era. In the ICS timescale, the Pleistocene is divided into four stages or ages, the Gelasian, Calabrian, Middle Pleistocene (unofficially the 'Chibanian') and Upper Pleistocene (unofficially the 'Tarantian'). In addition to this international subdivision, various regional subdivisions are often used.
Before a change finally confirmed in 2009 by the International Union of Geological Sciences, the time boundary between the Pleistocene and the preceding Pliocene was regarded as being at 1.806 million years Before Present (BP), as opposed to the currently accepted 2.588 million years BP: publications from the preceding years may use either definition of the period.
.... Wikipedia
The Pleistocene is the geological epoch which lasted from about 2,588,000 to 11,700 years ago, spanning the world's most recent period of repeated glaciations. The end of the Pleistocene corresponds with the end of the last glacial period and also with the end of the Paleolithic age used in archaeology.
The Pleistocene is the first epoch of the Quaternary Period or sixth epoch of the Cenozoic Era. In the ICS timescale, the Pleistocene is divided into four stages or ages, the Gelasian, Calabrian, Middle Pleistocene (unofficially the 'Chibanian') and Upper Pleistocene (unofficially the 'Tarantian'). In addition to this international subdivision, various regional subdivisions are often used.
Before a change finally confirmed in 2009 by the International Union of Geological Sciences, the time boundary between the Pleistocene and the preceding Pliocene was regarded as being at 1.806 million years Before Present (BP), as opposed to the currently accepted 2.588 million years BP: publications from the preceding years may use either definition of the period.
.... Wikipedia
~ The San Andreas Fault ~
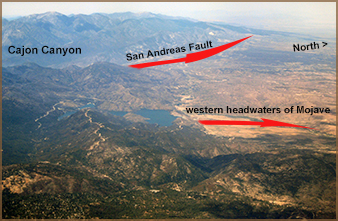
View over the Cajon Pass portion of the Transverse range facing westward. The San Andreas bisects the Transverse and creates the Cajon Pass. The fault then extends in a northwest direction.
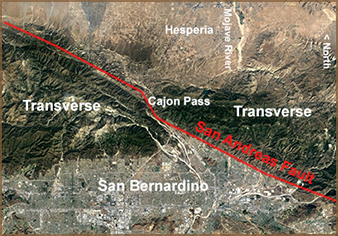
The San Andreas Fault before creating the Cajon Pass complex moves on a northwest path through the San Bernardino area, cuts through the Transverse Range and then continues northwest.
The San Andreas Fault is a continental transform fault that extends roughly 1,200 kilometers (750 mi) through California.[1] It forms the tectonic boundary between the Pacific Plate and the North American Plate, and its motion is right-lateral strike-slip (horizontal). The fault divides into three segments, each with different characteristics and a different degree of earthquake risk. The slip rate along the fault ranges from 20 to 35 mm (0.79 to 1.38 in)/yr.
The San Andreas began to form in the mid Cenozoic about 30 Mya (million years ago). At this time, a spreading center between the Pacific Plate and the Farallon Plate (which is now mostly subducted, with remnants including the Juan de Fuca Plate, Rivera Plate, Cocos Plate, and the Nazca Plate) was beginning to reach the subduction zone off the western coast of North America. As the relative motion between the Pacific and North American Plates was different from the relative motion between the Farallon and North American Plates, the spreading ridge began to be "subducted", creating a new relative motion and a new style of deformation along the plate boundaries. These geological features are what are chiefly seen along San Andreas Fault. It also includes a possible driver for the deformation of the Basin and Range, separation of the Baja California Peninsula, and rotation of the Transverse Range.
The southern segment (also known as the Mojave segment) begins near Bombay Beach, California. Box Canyon, near the Salton Sea, contains upturned strata associated with that section of the fault. The fault then runs along the southern base of the San Bernardino Mountains, crosses through the Cajon Pass and continues northwest along the northern base of the San Gabriel Mountains. These mountains are a result of movement along the San Andreas Fault and are commonly called the Transverse Range.
...Wikipedia
The San Andreas began to form in the mid Cenozoic about 30 Mya (million years ago). At this time, a spreading center between the Pacific Plate and the Farallon Plate (which is now mostly subducted, with remnants including the Juan de Fuca Plate, Rivera Plate, Cocos Plate, and the Nazca Plate) was beginning to reach the subduction zone off the western coast of North America. As the relative motion between the Pacific and North American Plates was different from the relative motion between the Farallon and North American Plates, the spreading ridge began to be "subducted", creating a new relative motion and a new style of deformation along the plate boundaries. These geological features are what are chiefly seen along San Andreas Fault. It also includes a possible driver for the deformation of the Basin and Range, separation of the Baja California Peninsula, and rotation of the Transverse Range.
The southern segment (also known as the Mojave segment) begins near Bombay Beach, California. Box Canyon, near the Salton Sea, contains upturned strata associated with that section of the fault. The fault then runs along the southern base of the San Bernardino Mountains, crosses through the Cajon Pass and continues northwest along the northern base of the San Gabriel Mountains. These mountains are a result of movement along the San Andreas Fault and are commonly called the Transverse Range.
...Wikipedia
~ The Transverse Ranges ~
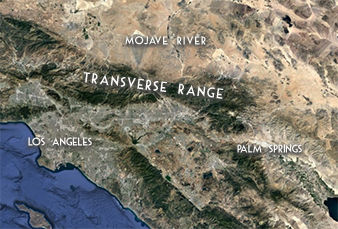
The Transverse Ranges are a group of mountain ranges of southern California, in the Pacific Coast Ranges physiographic region in North America. The Transverse Ranges begin at the southern end of the California Coast Ranges and lie between Santa Barbara and San Diego counties. They derive the name Transverse Ranges due to their east–west orientation, making them transverse to the general north–south orientation of most of California's coastal mountains.
Most of the Transverse Ranges are bounded to the north and east by the San Andreas Fault, which separates the ranges from the California Coast Ranges and the Peninsular Ranges. Notable passes along the fault include Tejon Pass, Cajon Pass, and San Gorgonio Pass. Components of Transverse Ranges to the north of the fault include the Tehachapi Mountains and the San Bernardino Mountains. The western and southern boundaries are generally acknowledged to be the Pacific Ocean and various alluvial valleys and basins. Major passes not along the San Andreas Fault include Gaviota Pass, San Marcos Pass, the Conejo Grade, Newhall Pass, and Cahuenga Pass.
...Wikipedia
Most of the Transverse Ranges are bounded to the north and east by the San Andreas Fault, which separates the ranges from the California Coast Ranges and the Peninsular Ranges. Notable passes along the fault include Tejon Pass, Cajon Pass, and San Gorgonio Pass. Components of Transverse Ranges to the north of the fault include the Tehachapi Mountains and the San Bernardino Mountains. The western and southern boundaries are generally acknowledged to be the Pacific Ocean and various alluvial valleys and basins. Major passes not along the San Andreas Fault include Gaviota Pass, San Marcos Pass, the Conejo Grade, Newhall Pass, and Cahuenga Pass.
...Wikipedia
~ Pleistocene Glaciation ~
The Quaternary glaciation, also known as the Pleistocene glaciation, is an alternating series of glacial and interglacial periods during the Quaternary period that began 2.58 Ma (million years ago), and is ongoing. Although geologists describe the entire time period as an "ice age", in popular culture the term "ice age" is usually associated with just the most recent glacial period. Since earth still has ice sheets, geologists consider the Quaternary glaciation to be ongoing, with earth now experiencing an interglacial period.
During the Quaternary glaciation, ice sheets appeared. During glacial periods they expanded, and during interglacial periods they contracted. Since the end of the last glacial period the only surviving ice sheets are the Antarctic and Greenland ice sheets. Other ice sheets, such as the Laurentide ice sheet, formed during glacial periods and completely disappeared during interglacials.
During the Quaternary glaciation, ice sheets appeared. During glacial periods they expanded, and during interglacial periods they contracted. Since the end of the last glacial period the only surviving ice sheets are the Antarctic and Greenland ice sheets. Other ice sheets, such as the Laurentide ice sheet, formed during glacial periods and completely disappeared during interglacials.
The major effects of the Quatenary glaciation have been the erosion of land and the deposition of material, both over large parts of the continents; the modification of river systems; the creation of millions of lakes, including the development of pluvial lakes far from the ice margins; changes in sea level; the isostatic adjustment of the Earth's crust; flooding; and abnormal winds. The ice sheets themselves, by raising the albedo (the extent to which the radiant energy of the Sun is reflected from Earth) created significant feedback to further cool the climate. These effects have been reshaping entire environments on land and in the oceans, and their associated biological communities.
With Northern Hemisphere glaciation during the Last Glacial Maximum the creation of 3 to 4 km (1.9 to 2.5 mi) thick ice sheets equate to a global sea level drop of about 120 m (390 ft).
Before the quaternary glaciation, land-based ice appeared, and then disappeared, at least four other times.
...Wikipedia
With Northern Hemisphere glaciation during the Last Glacial Maximum the creation of 3 to 4 km (1.9 to 2.5 mi) thick ice sheets equate to a global sea level drop of about 120 m (390 ft).
Before the quaternary glaciation, land-based ice appeared, and then disappeared, at least four other times.
...Wikipedia
~ The Mojave River and Associated Lakes ~
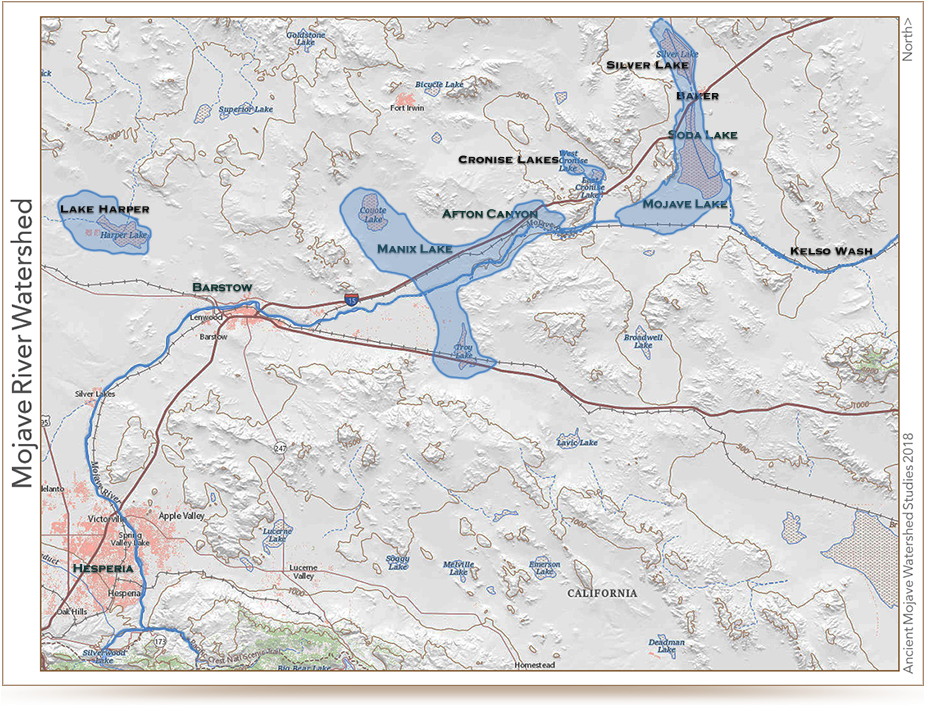
The Mojave River is the largest drainage system in the Mojave Desert. It's modern extent and capacity is only a fraction compared to its extent during the Last Glacial Maximum. At its peak during this last ice age, the Mojave River drainage basin extended from the San Bernardino Mountains in the west; it flowed east and north ultimately merging with the Amargosa River before draining into Lake Manley in Death Valley. At this peak period, waters of the Mojave River drainage system flowed through, or contributed water to, several great Pleistocene Lakes: Lake Manix (which incorporated modern dry lake basins Afton, Troy, Coyote, Harper, and Cronese basins), and Lake Mojave (including dry Soda Lake and dry Silver Lake basins)(see the map on the Changing Climates & Ancient Lakes page). Today Soda Lake is the current terminal point of the Mojave River (although it has flowed into Silver Lake in historic times).
This drainage system evolved along with the changing landscape beginning in late Tertiary time when concurrent tectonic uplift of mountain ranges around the Mojave region and changes in regional climatic conditions were occurring. The modern river system began developing as westward-flowing stream drainages were blocked by the uplift of the Transverse Ranges along the greater San Andreas Fault System. The combination of blocked drainage systems and increased precipitation with the onset of cooler or ice age conditions at the close of the Tertiary resulted in the filling of basins with water (and sediments). Progressively through the latest Tertiary and into the Quaternary periods, lakes filled and stream overflowed through low divides between ranges and flooded adjacent basins. In this manner, the Mojave River evolved from the spilling over of lakes in the western Mojave Desert region. These large lakes do not exist today. Two large lakes that played perhaps a most significant role in the development of the landscape in the Mojave National Preserve area were Lake Manix and Lake Mojave. Sediments associated with these ancient lake deposits (and others in the region) record a story of climate change in the region.
Lake Manix was a large inland lake that was located in the Barstow, CA region (dry Troy Lake and Coyote Lake are remnants of this larger lake basin), and Mojave Lake in the Baker, CA region (dry Silver Lake and Soda Lake are remnants of this ancient lake basin). The development of these lakes and other lakes in the region was progressive with time, with Manix Lake filling first, and Mojave Lake forming later when the Lake Manix filled to capacity and spilled westward, ultimately carving Afton Canyon during the last glacial maximum about 18,000 years ago (Jefferson, 2003).
Debate remains whether earlier in the Quaternary the greater Mojave River drainage system (including Lake Manley and the Owens River drainage) filled to overflowing capacity and spilled into the Bristol Lake basin (south of the Mojave National Preserve), or possibly to the Colorado River (Enzel et al., 2003, Cox et al., 2003; Anderson & Wells, 2003). No water from the Mojave River system flows into the Bristol Lake basin at present or in the recent past. And in general, groundwater drainage systems follow patterns in surficial flow, but whether groundwater originating from the Mojave River basin ever makes it to the Colorado River is not resolved.
This drainage system evolved along with the changing landscape beginning in late Tertiary time when concurrent tectonic uplift of mountain ranges around the Mojave region and changes in regional climatic conditions were occurring. The modern river system began developing as westward-flowing stream drainages were blocked by the uplift of the Transverse Ranges along the greater San Andreas Fault System. The combination of blocked drainage systems and increased precipitation with the onset of cooler or ice age conditions at the close of the Tertiary resulted in the filling of basins with water (and sediments). Progressively through the latest Tertiary and into the Quaternary periods, lakes filled and stream overflowed through low divides between ranges and flooded adjacent basins. In this manner, the Mojave River evolved from the spilling over of lakes in the western Mojave Desert region. These large lakes do not exist today. Two large lakes that played perhaps a most significant role in the development of the landscape in the Mojave National Preserve area were Lake Manix and Lake Mojave. Sediments associated with these ancient lake deposits (and others in the region) record a story of climate change in the region.
Lake Manix was a large inland lake that was located in the Barstow, CA region (dry Troy Lake and Coyote Lake are remnants of this larger lake basin), and Mojave Lake in the Baker, CA region (dry Silver Lake and Soda Lake are remnants of this ancient lake basin). The development of these lakes and other lakes in the region was progressive with time, with Manix Lake filling first, and Mojave Lake forming later when the Lake Manix filled to capacity and spilled westward, ultimately carving Afton Canyon during the last glacial maximum about 18,000 years ago (Jefferson, 2003).
Debate remains whether earlier in the Quaternary the greater Mojave River drainage system (including Lake Manley and the Owens River drainage) filled to overflowing capacity and spilled into the Bristol Lake basin (south of the Mojave National Preserve), or possibly to the Colorado River (Enzel et al., 2003, Cox et al., 2003; Anderson & Wells, 2003). No water from the Mojave River system flows into the Bristol Lake basin at present or in the recent past. And in general, groundwater drainage systems follow patterns in surficial flow, but whether groundwater originating from the Mojave River basin ever makes it to the Colorado River is not resolved.
The synchronicity of lake formation with cooler and wetter climatic conditions in the Mojave region's past has been the target of abundant research from many angles and for many decades. Developing an understanding of climatic cycles (wet to dry) is fundamental to resolving changing landscape conditions (including plant cover, soil development, erosion, alluvial fan processes, playas and lakes, etc.); and understanding the relationships between these different variable elements. For instance, Soda Lake is now essentially a dry lake basin (a playa), of which only temporary flooding occurs on roughly a decade basis. Currently, the Soda Lake basin is fed by groundwater flow and episodic stream discharge from the surrounding mountain ranges and high-flow discharge from the Mojave River. Enzel et al. (2003) suggests that the average annual flow of the lower reaches of the Mojave River would have to be nearly a magnitude larger than the river's average current annual flow of 9,500,000 cubic meters. This 10-times volume would be necessary, along with reduced evaporative conditions, to maintain a lake consistent with the last glacial maximum. This means that rainfall amounts would probably need to be several times greater than current regional averages, and seasonal cooling and humidity would need to be significantly greater and last longer to overcompensate for evaporation effects. Currently, evaporative potential in the basin regions exceeds stream and groundwater flow input, and hence, today no lakes exist and desert conditions prevail.
The linkage of climatic changes with surface processes is only partly understood. There is undetermined lag between when cool and wet conditions start and when lakes can form and fill to their capacity. Also, the timing between the start of cool and wet conditions and the when ecological communities adapt and spread across the region may not not be synchronous. The reverse is true for changes from wet to dry conditions. Regional lake deposits show drying occurred about 9,000 years ago as sedimentary records transition from lake deposits, to intermediate marshland conditions, and to dry and desiccated playa conditions. The change from wet to dry conditions had a major impact on the ecology of the Mojave region (the vegetative cover and the animal species the landscape supported, including humans). Plant communities that were probably prevalent throughout the lower landscape regions are now restricted to the highest and wettest mountain areas, and probably many other species existed in the region that were adapted to a completely different seasonal pattern, but do not exist today. Likewise, aquatic or semi-aquatic species (fish, amphibians, turtles, arthropods, etc.) adapted to lake environments are restricted to spring-fed ponds or ephemeral spring fed streams, such as in portions of the Mojave River in Afton Canyon. The distribution of these species across the Mojave region (including in the Colorado River) may require a greater regional drainage system in prehistoric times.
...US Geologic Survey
The linkage of climatic changes with surface processes is only partly understood. There is undetermined lag between when cool and wet conditions start and when lakes can form and fill to their capacity. Also, the timing between the start of cool and wet conditions and the when ecological communities adapt and spread across the region may not not be synchronous. The reverse is true for changes from wet to dry conditions. Regional lake deposits show drying occurred about 9,000 years ago as sedimentary records transition from lake deposits, to intermediate marshland conditions, and to dry and desiccated playa conditions. The change from wet to dry conditions had a major impact on the ecology of the Mojave region (the vegetative cover and the animal species the landscape supported, including humans). Plant communities that were probably prevalent throughout the lower landscape regions are now restricted to the highest and wettest mountain areas, and probably many other species existed in the region that were adapted to a completely different seasonal pattern, but do not exist today. Likewise, aquatic or semi-aquatic species (fish, amphibians, turtles, arthropods, etc.) adapted to lake environments are restricted to spring-fed ponds or ephemeral spring fed streams, such as in portions of the Mojave River in Afton Canyon. The distribution of these species across the Mojave region (including in the Colorado River) may require a greater regional drainage system in prehistoric times.
...US Geologic Survey
~ Pluvial Lakes ~
A pluvial lake is a body of water that accumulated in a basin because of a greater moisture availability resulting from changes in temperature and/or precipitation. These intervals of greater moisture availability are not always contemporaneous with glacial periods. Pluvial lakes are typically closed lakes that occupied endorheic basins. Pluvial lakes that have since evaporated and dried out may also be referred to as paleolakes.
Geology ~ Pluvial lakes represent changes in the hydrological cycle: wet cycles generate large lakes, and dry cycles cause the lakes to recede. Accumulated sediments show the variation in water level. During glacial periods, when the lake level is fairly high, mud sediments will settle out and be deposited. At times in between glaciers (interglacial), salt deposits may be present because of the arid climate and the evaporation of lakewater.
Several pluvial lakes formed in what is now the southwestern United States during the glaciation of the late Pleistocene.
Geology ~ Pluvial lakes represent changes in the hydrological cycle: wet cycles generate large lakes, and dry cycles cause the lakes to recede. Accumulated sediments show the variation in water level. During glacial periods, when the lake level is fairly high, mud sediments will settle out and be deposited. At times in between glaciers (interglacial), salt deposits may be present because of the arid climate and the evaporation of lakewater.
Several pluvial lakes formed in what is now the southwestern United States during the glaciation of the late Pleistocene.
One of these was Lake Bonneville in western Utah, which covered roughly 19,000 square miles (49,000 km2). When Lake Bonneville was at its maximum water level, it was 1,000 feet (300 m) higher than the Great Salt Lake.
Fresh water mollusks have been found in mud deposits from Searles Lake in California and suggest that the water temperature was about 7 degrees Fahrenheit (or 4 degrees Celsius) cooler than current temperatures. Radiocarbon dating of the youngest mud beds yield dates from 24,000 to 12,000 years ago.
Formation ~ When warm air from arid regions meets chilled air from glaciers, cloudy, cool, rainy weather is created beyond the terminus of the glacier. That humid climate was present during the last glacial period in North America and caused more precipitation than evaporation. The increase in rainfall fills the drainage basin and forms a lake.
During interglacial periods, the climate becomes arid once more and causes the lakes to evaporate and dry up.
...Wikipedia
Fresh water mollusks have been found in mud deposits from Searles Lake in California and suggest that the water temperature was about 7 degrees Fahrenheit (or 4 degrees Celsius) cooler than current temperatures. Radiocarbon dating of the youngest mud beds yield dates from 24,000 to 12,000 years ago.
Formation ~ When warm air from arid regions meets chilled air from glaciers, cloudy, cool, rainy weather is created beyond the terminus of the glacier. That humid climate was present during the last glacial period in North America and caused more precipitation than evaporation. The increase in rainfall fills the drainage basin and forms a lake.
During interglacial periods, the climate becomes arid once more and causes the lakes to evaporate and dry up.
...Wikipedia